Tuesday, February 28, 2012
Thursday, February 23, 2012
The Parable of the Garden
(From the flash reading CSFW did at Boskone.)
It was pretty sweet in the garden. No winter. Lots of food. Adam and Eve lying around naked-- not that they knew they were naked, of course. That came later. There was death but nobody knew what it was.
Being first, Adam wasn't all there. He walked around like an idiot naming everything. It wasn't his fault. His brain was packed in grease when he woke up. It was years before it worked right. Eve was smart right off, being human 2.0.
But like any monkey with an oversized brain and not much to do, both of them got bored. Adam less than Eve since he spent a lot of time figuring out how to name things. Should the word reflect the sound of the thing? The smell? What about things that looked like a combination? Like an elephant: snake on both ends and a hippo in between. Even Adam had a few doubts about that one.
Eve, created unstupid, found it much harder to keep herself entertained. The obvious solution never occurred to her. Adam and Eve had the equipment for sex but not the skills or motivation.
In the middle of the garden was the tree. You know the one. A lot of the stories have two trees, the other being the tree of life. But you can't really imagine anybody would leave immortality just lying around, would you? As it was, there were worms, flies and fruit bats filled with the knowledge of Good and Evil, copulating wildly around the tree in a pointless effort to relieve the depression of their inevitable mortal demise.
There wasn't much variety in the garden.
Eve was walking around staring at the same maple (Look, Eve! A Maple!), fig (Look, Eve! A Fig!) and cranberry (Look Eve... You get the idea.) until she was ready to scream. If they'd invented fire at that point Eve would have burned the garden to the ground and happily danced in the ashes. It's not surprising she'd gravitate towards the forbidden.
The snake actually lived in the tree. Being off limits, it was the one place in the garden he could find privacy. (Look, Eve! A Snake!... Ow. My heel.) The serpent was cursed with a good understanding of things to come (Thou shalt crawl on thy belly on the ground! Well, duh! No arms!) and was none too keen on his prospects.
"Hi," said Eve.
The snake nodded.
"So," she says. "Is there anything to do around here?"
The snake shrugged. (Something to see for someone without arms.) "There are always these apples."
"Those are forbidden."
"Suit yourself."
"What's so special about them?"
"Knowledge of Good and Evil."
"So?"
The snake sighed. "With good and evil you get the rest of the package: tight dresses, moral decisions, scientific inquiry, multiple orgasms."
"Beg pardon?"
At the point the snake realized how truly bereft these two ignorant mammals were and gave Eve a sample. From what we know about the snake it was, no doubt, a pretty good sample.
When Eve could breathe again she had an inkling of what was at stake.
"I'd be naked," she said, scandalized and excited at the same time.
"More to the point," said the snake. "You would know what nakedness was for."
Eve bit into the apple without hesitation.
Wednesday, February 22, 2012
The Parable of the Thief
(From the flash reading CSFW did at Boskone.)
Once there lived a thief of extraordinary deftness. His hands were so quick at deciphering the quality of a lock that it seemed that he had only to brush his fingers against the metal and the tumblers would open of their own accord. He grew so accustomed to his skill that it seemed to him that the skill of his hands were embedded in their sinew and bone rather than deriving from his mind and heart.
Indeed, his hands were so skillful and brilliant that they developed their own awareness and sophistication in perceiving the world. The right one observed how it was being utilized and in what manner. It attempted to discuss these activities with the left hand, but the left hand had its own nature kept its own counsel.
Over time, the right hand developed its own moral sense and purpose. It developed a conscience. The use of its considerable gifts in the service of thievery disturbed it. For a long time, it allowed itself to be used in this manner, against its own now deeply held beliefs, but too doubtful to criticize.
Eventually, the right hand decided it had had enough and refused further business of this sort. It made a fist when employed against a lock. Stiffened when soft and supple fingers were required.
This enraged the thief and he sat down and rebuked the hand, saying, "You must do as I command."
The hand, though frightened, spoke up: "I can see what you're doing is wrong and offensive. I will not serve in this matter further."
The thief considered this. He said: "Where were you when I was first learning to touch and hold things? Did you not remain silent? Were you not stupid from ignorance? Where were you when I touched a woman or caressed a flower? Did I not teach you suppleness and daring? Did I not give you opportunities to gain your own conscience? All that you are derives from me-- even your conscience and reluctance. Your thoughts are my thoughts. Your impulses are my impulses. Your deftness is my own. If your lesser intelligence is to be of any use in the world, it must be allied with my greater one."
The hand considered this and decided that the thief was right. It would remain silent and submit to the thief's will as a higher order being.
With that, the thief resumed his ways.
In time, the thief was captured and given punishment. In those days, this consisted of the amputation of his right hand. The hand, of course, withered and died. Forever after the thief tearfully cried in the middle of the night, mourning his loss to his left hand and lamenting his fate. Occasionally, he cursed the left hand, saying "Why did you not try to stop me from my ways as did your brother? With both of you against me, I would have had no choice but to change my ways."
But the left hand had its own nature and kept its own counsel.
Sunday, February 19, 2012
Biological Revolutions: The Prokaryotes Cometh
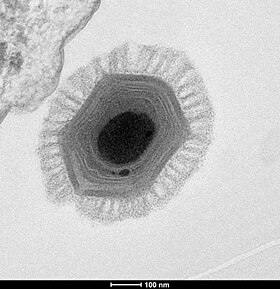
(Picture from here.)
Last time we talked about nothing less than the origin of life.
Seems like everything ought to be downhill from that, eh?
"Life" as we defined it consisted of self-replicating metabolizing cellular systems. But what we came up with didn't much resemble life we we know it today. Inheritance was via RNA and not DNA. Catalysis was by RNA enzymes-- ribozymes-- with some proteins. No photosynthesis and nowhere near as complex a system-- or ecology-- as we see today.
So: there are to aspects to the prokaryote revolution: 1) how did it happen? and 2) what did it do to the world? We'll deal with part 2 in another post.
As to how the prokaryotes first came to be we have to think a little about what prokaryotes are.
Currently we define life as being divided into three domains: Bacteria, Archaea and Eucaryotes. Eucaryotes have nuclei containing DNA in the cells. In fact, eucaryotes have lots of little organelles inside them: nuclei, mitochondria, chloroplasts and Golgi apparatus, among others. These are all referred to as membrane bound organelles in that they have their own membrane structures that either are their own or are internal reflections of the cells own membrane. We belong to the Eucaryota and therefore lump all things not us in their own group, the Prokaryotes.
This is analogous to the old way we defined animals as vertebrates (us) and invertebrates (not us.)
However, there is some utility in this discussion in drawing together the Bacteria and Archaea as prokaryotes. The current evidence suggests that something prokaryotic lived 3.8 billion years ago. Eucaryotes are comparative newcomers and appeared only six hundred million years ago. The first life forms appear to be prokaryotes. So the bridge from the RNA world to the Last Universal Common Ancestor (LUCA) and to us appears to have involved the development of the prokaryotes.
Prokaryotes don't carry the baggage of the organelles. Their DNA, ribosomes, enzymes, etc., are more exposed. Prokaryotes have a single loop of stabilized DNA that is stored in something called a nucleoid and is not tightly bound in chromosomes. There may be more than one copy of the DNA existing in the cell at a given time.
Patrick Forterre has delved deep into this area and come up with some very interesting speculations. While I don't think all of his conclusions are supported (viruses being living things is one, for example) he makes some interesting arguments.
Living or not, we know that viral particles are the most abundant biological entities in the current biosphere. They are ten times more abundant than the bacteria in the upper ocean, for example. (See here.) This shouldn't be surprising with a little thought. The ratio of produced virus particles to infected cell is huge. To pick a number of, say, 100k particles/infected cell and an infection rate in a population of, say, 1/10k cells, it would only take 1 cell in 10,000 cells to maintain that ratio. Some viruses replicate and several times that amount and some viruses have a much higher infection rate. Then, there are all those cells dying for lots of reasons. Some of which contain virus particles in their make up.
Forterre suggests this is not a new circumstance. It must also have been true at the time of LUCA. In fact, pretty much as soon as there were cellular organisms with replicating systems were were biological agents (viruses) ready and willing to hijack said systems. So viruses have been with us for a long time.
Viruses also infect all three domains of organisms and with a plethora of different mechanisms. Viruses are also biochemically more related to each other than the cells they infect. Most of their proteins have no specific relationship with their hosts and there are many families of viruses infecting different hosts but produce similar proteins to each other. Viruses, then, show a different biochemical signature from modern organisms.
Viral biochemistry has to come from somewhere. Forterre suggests they came from biochemistry that predates the modern world. Hence, RNA viruses might well be the last relics of the RNA world. DNA viruses might have been instrumental in the creation of the DNA world.
How's that again?
Well, how does a virus work? It has a seed form, a "virion", that attaches to the cell membrane and allows the insertion of a mix of inheritable material (RNA or DNA) and proteins into the cell. That material preps itself-- different viruses do this step different ways-- until it is in a position to make more viruses. Some viruses create actual virus factories that then produce viruses. Others subvert existing machinery to achieve a similar end. At some point the cell extrudes the virus. This can be as simple as the cell exploding ("lysing") or may be a more complex mechanism that leaves the cell membrane intact.
An interruption of any one of these cells can leave the cell in a new state where there is additional protein machinery in place and new heritable material. There's not much intrinsic difference between a DNA strand of a virus and a DNA strand of E. coli. They have genes. They have start and stop codons. Some strands can be recognized as "other" and destroyed and some can't. We've seen-- heck, we've used-- this incorporation of virus DNA into the cell DNA. That's how genetic engineering is often done. What can be done in the lab is often been done before in the field.
Some virus families that parasitize Archaea, Bacteria and Eucaryota have enough common ground between them to suggest they evolved from an ancestor that predated LUCA.
DNA is harder to manipulate than RNA. So there needed to be complex proteins available before it could be used. RNA has no such issue since RNA by itself has enzymatic activity. So the DNA world could not have happened without a prior ancestor that was not DNA dependent. And finally, we need to somehow bring proteins into this process.
If LUCA used DNA for inheritance, used RNA to encode proteins and used RNA and proteins to catalyze processes, that organization had to come from somewhere. And, since heritage is all in biology, something had to have it first and propagate it. Viruses have some neat potential solutions to these problems.
For one thing, viruses drive down enormously the cost of change. Cells must create other cells. They are discrete entities. Loss of that unit organization is synonymous with death. Viruses do not have that limitation. They are concerned with encapsulating an infectious capsid. For the capsid's contents to survive it need only find a compatible cells. Since cells are fighting back, it is in the interest of virus to mix it up as much as possible.
Consequently, viruses inherently evolve more quickly and take more chances in their evolution than cell based system. In addition, replication is a dirty business with lots of leftover bits and mistakes. Cells invest in systems to prevent these. Viruses have no such incentive-- to them the mistakes are opportunities to try something new. Hey, if thirty percent of your capsids aren't infective, who cares? There's seventy thousand that are and a lot of them are different. (See here and here.)
So. Here we have an RNA world with a sea of viruses and cells. Lots of variation in both cells and viruses. Some viruses are capturing RNA from their hosts and propagating it. Some viruses are injecting new RNA into their cells and the cells capture it. Some are doing both. Cells themselves are variable in construction. Some use lipid membranes. Some membranes are inorganic. Some chemical reactions might be taking place outside of cells entirely. Everything is exchanging with everything else-- much more than now. Even then, however, capsid exchange is inherently more rapid than cellular exchange. The whole genome pool is parasitic on itself.
For example, consider a capsid containing protein production machinery (encoded in RNA) injects itself into a cell that uses ribozymes. Let's say it's defective; it can't encode other capsids. But the machinery itself can metabolize. This cell now has a selective advantage of neighboring cells that only use ribozymes. That cell might absorb/digest/exchange the encoding of a membrane. The chemistry doesn't care. It produces membrane.
Large viruses (such as the mimivirus) have biochemical systems that rival the size of biochemical systems of their host. The viral factory of the mimivirus is nearly as large as the nucleus of its amoeba host. (see here.) One wonders if, long ago, a similarly large virus infected a host and stayed there, its biochemical pathways replacing those of its host. Eventually, the cell divides and it's the virus genome, not the original host, that is preserved.
At some point, some members of this ancient pool become organized. Genes that encode replication are linked to genes that encode accessory functions such as metabolism. Expression of that encoding (such as proteins) become more uniform as a result. At this point the cooperative nature of the primordial pool becomes competitive as something resembling cell lines appear. It's to the advantage of a cell line to propagate itself and compete with other systems and cell lines for resources. What had been more of a chemical selection becomes natural selection and both cells and capsids participate.
All of this could have happened without DNA. The RNA world could have become quite complex. Fast cellular evolution. Faster virus evolution. The RNA cellular fights back and the virus has to change in order to remain competitive.
DNA has a greater replication fidelity than RNA-- it's a much less noisy chemical reaction. A virus that changed the transported RNA into DNA as part of the infection process is more assured of retaining identity while under attack by cellular defense mechanisms. When this first happened it would be a powerful tool since the DNA would not necessarily even be recognized as dangerous material by cellular defenses. Like many virally induced changes, this could have been incorporated in the cellular genome. (See here.)
Using DNA instead of RNA slows the pace of evolution while it increases constancy across generations.
These sorts of things happened not once but many times. Eventually, a cell line stayed viable and became honed through natural selection into LUCA. After LUCA, exchange of DNA slowed and out of those cell lines arose something resembling species-- though the concept is blurry and vague at this point.
It doesn't matter. The prokaryotes were born.
They were about to take over the world.
Cited works and additional reading:
- Defining Life: The Virus Viewpoint, Patrick Forterre
- The origin of viruses and their possible roles in major evolutionary transitions, Patrick Forterre
- The ancient Virus World and the evolution of cells, Koonin, Senkevich and Dolja
- Giant Viruses: Conflicts in Revisiting the Virus Concept, Patrick Forterre
- Mimivirus: the emerging paradox of quasi-autonomous viruses, Claverie and Abergel
- A new fusion hypothesis for the origin of Eukarya: better than previous ones, but probably also wrong, Patrick Forterre
- Origin and evolution of DNA topoisomerases, Patrick Forterre
- On the last common ancestor and early evolution of eukaryotes: reconstructing the history of mitochondrial ribosomes, Desmond, Brochier-Armanet, Forterre, Gribaldo
- The origin of eukaryotes and their relationship with the Archaea: are we at a phylogenomic impasse?, Gribaldo, Poole, Daubin, Forterre, Brochier-Armanet
- Bacteria with a eukaryotic touch: A glimpse of ancient evolution? Forterre, Gribaldoa
- The Interplay between RNA and DNA Modifications: Back to the RNA World, Forterre, Grosjean
- The two ages of the RNA world, and the transition to the DNA world: a story of viruses and cells, Patrick Forterre
Friday, February 17, 2012
Thursday, February 9, 2012
Sunday, February 5, 2012
Biological Revolutions: Abiogenesis Part 2

(Picture from here.)
This is the follow on to the abiogenesis entry I did here. There we talked for probably much too long about containment and how crucial it was to derive modern organisms. Today we're going to talk about the subject dear to our hearts: Okay. We've got a sack for the protoplasm. What the heck are we going to put in it.

Remember the four prerequisites we said we needed to overcome to get to life as we know it today:

Remember the four prerequisites we said we needed to overcome to get to life as we know it today:
- Containment: creating the enclosed environment in which the chemical processes can occur. Living systems use a cell for this.
- Metabolism: deriving additional material by using energy producing chemical reactions to power energy requiring chemical reactions. Living systems use proteins and, to a limited extent, RNA to do this.
- Inheritance: one chemical system has to be able to drive the creation of other chemical systems. Living systems do this by reproduction involving DNA.
- Coupling: This joins containment, metabolism and inheritance into a package. Inheritance doesn’t do any good if you’re not inheriting the ability to metabolize. The whole DNA to RNA to Protein complex is how living systems do it.
We talked about containment last time and we can't talk about coupling until we talk about metabolism and inheritance. So. Now we'll talk about metabolism and inheritance.
There are essentially two approaches to abiogenesis models. These are called metabolism first models and replication first models. Historically, approaches to metabolism were first proposed and more recently replication has been making the rounds. We'll start with metabolism.
Metabolism First
J. B. S. Haldane was the first one to suggest that the prebiotic oceans would be significantly different from modern oceans. But in 1952, the Miller-Urey experiment brought it home. In short, Miller and Urey brought together a mixture of water, hydrogen, methane and ammonia and cycled it through a system that periodically sent sparks through it. In short order, they found various interesting biochemical compounds, notably amino acids, were present. This gave experimental heft to the idea. Consequently, several biochemical models of metabolism were then proposed.
Metabolism is, in short, the reproducible extraction of energy from high energy sources (chemical, light, etc.) to produce complex organic molecules. Plants use light to take CO2 and turn it into hydrocarbons that can be used by the plants. Some archae use sulfides produced by geochemical reactions. We take the products of plants or other animals and use them as our high energy sources and produce our own organic molecules. While the high energy source is variable, what must be done is fairly clear. We need to get to a metabolism of sorts and from that metabolism to our metabolism.
All living systems use variants of fermentation and the Kreb cycle to get energy from high energy carbon sources. Plants and sulfur loving bacteria add the step of grabbing a CO2 to get the energy but once they get that high energy carbon compound they use it in a similar fashion.
Any origin story of metabolism has to start with the building up of organic molecules as a consequence of chemical reactions that come from a non-biological origin. The problem to be overcome by all of these models is to create long chain molecules that don't automatically self-hydrolyze back to their constituents. By analogy, spinning a disk doesn't help us since the disk can spin in both directions. What we need is a ratchet that only spins one way. Or, at least spins in one way statistically.
There are a lot of models but I'm only going to talk about three: Eigen's hypercycle model, the iron-sulfur world and thermogenesis.
A hypercycle is a collection of interacting chemical cycles, each of which operate independently. Each of the chemical cycles is self-reinforcing loop and the aggregate represents a net gain in chemical complexity. (Eigen and Schuster's 1978 article is here.) The target of a hypercycle is a quasispecies. This is a collection of cycles that reproduce themselves. In this case, while the metabolism is inherited what we normally think of as reproduction doesn't apply. A hypercycle in a microsphere that grew large enough to break apart into two microspheres, each of which had a full complement of the hypercycle would satisfy the concept.
One way to visualize a hypercycle is as a collection of differently shaped and sized gears. Each gear represents its own little cycle. But the output of one is the input of another. The problem with Eigen and Schuster's original hypothesis was that they had to use nucleotides and proteins o create the process. As such their hypothesis couldn't explain the origin of nucleotides and proteins.
Enter Günter Wächtershäuser and the iron-sulfur world.
Wächtershäuser proposed that the pioneer organism lived in a volcanic hydrothermal flow. He suggested that mineral based catalytic chemistry could produce all of the polymerization required by abiogenesis. Note that the environment had an abundance of energetic iron and nickel as well as possible cobalt, manganese, tungsten and zinc.
For a moment recall that iron, manganese and zinc are all dietary trace elements in modern systems. Tungsten and cobalt are not. But tungsten is in chromium's column in the periodic table so there is some chemical similarity. Cobalt is not used by us but it is used by bacteria in vitamin B12 synthesis.
Wächtershäuser postulated that a sulfur based cycle that resembled the Krebs cycle was possible. Carbon monoxide, ammonia and hydrogen sulfide would pass over catalytic metal compounds under high heat and pressure. This results in the formation of catalytic metallic peptides. Which then can catalyze more interesting compounds. Several of these reactions have been demonstrated experimentally. In addition, it has the added advantage that many of these reactions can occur without cellular containment.
Imagine, for example, if these reactions were occurring in the wall of a hydrothermal vent. Possible in the pores. Possibly in an organic slime. At some further date, the reactions can be encapsulated in a microsphere.
Karo Michaelian went one better. He suggested that we are looking at living systems backwards. He wanted to analyze them on the basis thermodynamics. From that point of view, living systems are unit producers of entropy-- not a surprise, right? Since everything produces entropy. Starting there he suggested that entropy isn't just a byproduct of their chemical reactions; it's the reason for their existence. Living systems arose because they were more efficient at producing entropy than their competition. According to him, nothing much beyond thermodynamics was necessary to cause living systems. They were as natural in their environment as any other chemical reaction. (The original paper is here.)
As an example, he pointed out that RNA and DNA would have existed as chemical compounds pretty much as soon as the temperature of the ocean cooled enough. He pointed out that RNA and DNA both absorb and dissipate UV with a wavelength of about 200-300nm. Which, coincidentally, was the wavelength of UV that fell on the prebiotic earth.
Along with visible light being absorbed by other materials, at the ocean surface, about 1mm depth, such absorption and dissipation would raise the surface temperature of the water about 5-6 C. The temperature of the surface would change on a day/night cycle. RNA and DNA would denature during the higher temperatures of the day. Then, at night when the temperature dropped polymerization would occur using any remaining single stranded molecules as a template. Michaelian's chemistry isn't exactly metabolism and it isn't exactly replication. But it serves as segue to the replication first models.
Replication First
It was long felt that metabolism had to come first since 1) the reactions were less complex and 2) they didn't need RNA or DNA to operate. Both RNA and DNA are problematic. They're complex molecules that are fragile and finicky. It was thought that a more robust system could then incorporate replication later.
That was then. The RNA world is now.
The RNA world hypothesis proposes that the form of life that existed prior to the current form of life was based on ribonucleic acid. The RNA world is based on the proposition that while some nucleotides bind together and break apart, some combinations had catalytic properties that lowered the required energy of their chain's creation. This meant that when these bonds occurred, they were less likely to break apart. This manifested in polymerization of the nucleic acids into RNA. The most efficient molecules were those that best catalyzed their own creation-- opening the opportunity for natural selection of chemical systems. Such catalytic compounds have been found. They are enzymes of a sort and are called ribozymes.
Over time, competition between ribozymes can foster cooperation. Some develop catalytic properties that cause peptides to bind together into small proteins. Proteins are much more efficient enzymes than ribozymes at this and any RNA compound that can synthesize itself and an enzyme out of the soup would certainly have a selective advantage.
One step in protein synthesis, aminoacylation of RNA, has been demonstrated in a short RNA segment. Aminoacylation is one of the chemical reactions used in transfer-RNA and modern protein synthesis.
Coupling Replication and Metabolism
It's a common fallacy among people that there is a single source of things from which all other things derive. Scientists (who are human regardless what some may think) share this fallacy. Sometimes the fallacy is productive-- such as the long pursuit of a unified physics. Other places, such as biology, it often hurts our understanding. If we know anything about living systems and their chemistry it's that if something can be done it probably was. It's a sort of biological analogy of rule 34: if it can happen it did and there's probably porn for it.
So I suspect all of these models and hypotheses occurred at one point or another. Iron-sulfur thermongenic hypercycles ran for millions of years in the RNA world. My bet is on the RNA world since there appears to be a coupling mechanism that occurs as a side effect of a known reaction. But I have a soft spot for iron-sulfur. Just because the idea of all that chemistry in an undersea smoker is very very cool.
We do know is that it changed at some point to the world we now live in. If we had an RNA world it had to change tothe DNA one. So what happened.
Enter Patrick Forterre and his Biology of Extremophiles Laboratory. (Sounds like a band, doesn't it?)
Forterre has suggested that viruses were critical in the push from RNA based life to DNA based life. His hypothesis is that the Last Universal Common Ancestor was RNA based in a world of RNA viruses. Further, that some viruses began using DNA instead of RNA and were selected for given the inherent stabilities of DNA vs. RNA and the fact that the RNA world would only imperfectly defend itself from a DNA virus. But things Go Wrong and some of the DNA remains intact inside a cell filled with RNA and protein machinery.
Thus the prokaryote was born.
But that is a topic for another time.
Thursday, February 2, 2012
Profiteering on Cancer
Check out these articles on the Susan G Komen foundation. Then punt the pink ribbon.
Subscribe to:
Posts (Atom)